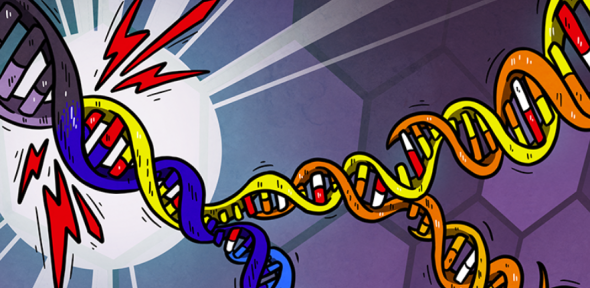
Submitted by Sophie Milbourne on Wed, 12/06/2024 - 16:59
From snowflakes and sunflowers, to butterflies and humans, symmetry is abundant throughout nature. There is also an elegant symmetry to the double helix structure of DNA – the blueprint of life. Yet, many of the DNA’s functions are asymmetrical: only one strand is needed for certain cellular processes, and some key proteins prefer one strand over the other.
One asymmetric process that is key to the function of DNA is replication, which occurs every time a cell divides. Using the two existing strands as templates, one new DNA strand is created as a long continuous string (the leading strand), whereas the other is synthesised as short sections which are subsequently linked together (the lagging strand). These vital steps allow genetic information to be accurately passed on during cell division.
DNA damage is a major cause of mutations, which is an early event in the development of many cancers. Our cells are exposed to damaging agents every day through the air we breathe, the food/drinks we consume, the UV light in the environment, and also some medicines such as chemotherapy drugs. Fortunately, most of this damage gets repaired before it becomes a permanent change, or ‘mutation’ in the DNA. However, when a mutation does occur, the wrong DNA base gets added, which can potentially change the ‘message’ that piece of DNA is coding. If this error is not repaired, then it gets passed on to all its daughter cells.
In a new paper published today, in the journal Nature, researchers from the Aitken lab at the MRC Toxicology Unit collaborated with the Taylor group at the MRC Human Genetics Unit in Edinburgh and other scientists around Europe. They chemically induced a single burst of DNA damage to liver tumours and mapped the resulting mutations to each of the two DNA strands to understand how the strand-asymmetric processes of DNA damage and repair influence the rate and distribution of mutations.
Dr Sarah Aitken, Group Leader at the MRC Toxicology Unit and co-senior author of this study said “Each time the DNA replicates there is both the opportunity for repair mechanisms to act, but also the risk of introducing mutations. Interestingly, we found that, despite the leading and lagging strand representing two distinct ways of copying DNA, there was a remarkable symmetry in mutation rate between the two DNA strands. This was unexpected because it is different to the patterns previously seen for other mutagens such as UV-induced DNA damage.”
Furthermore, DNA needs to be tightly wound and coiled up to fit into a cell’s nucleus. For certain cell functions, like transcription, the DNA needs to loosen a little and become more accessible. These ‘open’ regions tend to have fewer mutations, which could be due to either less damage occurring or more efficient repair of the damage. The researchers discovered that variation in mutation rate across the cell’s DNA is usually due to more efficient repair of the more accessible DNA, rather than differences in the amount of DNA damage.
Normally, the repair of any damage is very accurate and is completed before the cell divides. However, Anderson et al found that when there are clusters of DNA damage on both strands, then the usual repair machinery can actually create mutations. This is most likely to occur in regions of DNA that are most efficiently repaired, which also tend to be important control sites; like those that determine when a gene should be ‘switched on or off’. Any errors in the DNA at these sites can be particularly effective at turning an otherwise healthy and normal cell into one that becomes cancerous.
Dr Aitken, a CRUK Clinical Scientist, continued “Human cancer genomes represent a snapshot of the DNA landscape that has been damaged and incompletely repaired over a lifetime. Using our model system we have been able to start to disentangle these processes to better understand some of the very first steps in cancer development.”
Co-lead researcher Professor Martin Taylor from the MRC Human Genetics Unit added: “Understanding this could also help refine treatment with chemotherapeutics which themselves cause DNA damage, where it might help to minimise these especially important mutations caused by the repair.”
The work presented here forms part of a collaborative initiative by the Liver Cancer Evolution Consortium to understand how DNA damage and genetic background interact to mutate the genome and drive the development of cancer.
‘Strand-resolved mutagenicity of DNA damage and repair’ was published in the journal Nature on 12th June 2024. Read the full publication here.